Sterilizing immunity 101: "Sterilizing immunity: Understanding COVID-19" (Wahl et al.); this paper has some very useful primer details, refresher and I thank Dr. Ramin Oskoui for pointing it out
by Paul Alexander
The missing piece in this paper is the application of a sub-optimal vaccine (COVID vaccine) when there is circulating virus, high infectious pressure; they write disregarding the elephant in the room
SOURCE:
‘Immune memory develops during primary infections to protect from future exposures to the same pathogen. Vaccines mimic this response and induce immune memory that protects from severe disease and, in some cases, from symptomatic infection. If the pathogen is eliminated before it can replicate, natural and vaccine-induced immune memory can prevent the establishment of the infection, mediating sterilizing immunity. Sterilizing immunity protects the individual and prevents transmission to new hosts, thereby contributing to protection at a population level. Here, we describe the basic concepts of sterilizing immunity and discuss its relevance for protection in the context of SARS-CoV-2.’
‘Basic concepts underlying antibody-mediated immune protection
‘Acute immune responses are characterized by immediate inflammatory reactions through the innate immune system and the subsequent activation and expansion of B and T cells, which mediate slower but highly specific adaptive immunity. B cells produce serum antibodies, which mediate protection by marking pathogens for immune elimination and interfering with the invasion of host cells. T cells promote B cell responses and kill infected host cells. Upon clearance of the infection, the adaptive immune response contracts, allowing the immune system to remain flexible and able to react to new threats. Although the contraction phase is associated with a gradual decrease in serum antibody levels over time, long-lived plasma cells, which reside in special survival niches in the bone marrow, continue to produce pathogen-specific antibodies. The baseline serum antibody levels that are maintained long term by this B cell subset represent an immediate and highly potent defense mechanism upon re-exposure to the same pathogen.
Memory B cells that developed during the first infection or vaccine response help to increase antibody titers immediately in response to a second encounter with the same pathogen. Upon differentiation into antibody-secreting cells, memory B cells mediate a sharp rise in antibody titers within a few days. Because memory B cells and plasma cells have been selected to make antibodies that bind the pathogen more efficiently than their precursor cells, a process referred to as affinity maturation, the antibody response to re-infection is also of higher quality. Thus, because of the formation of immune memory, subsequent immune responses against the same pathogen are much faster and more potent, enabling the efficient elimination before the development of symptomatic (or at least severe) disease (Figure 2). However, re-exposures are often associated with antigenic diversity, e.g., when viruses accumulate mutations, as seen in the ongoing SARS-CoV-2 pandemic, or when we encounter members of bacterial species that differ in their surface antigen structures. Elimination of these variant pathogens is more difficult to achieve because high-affinity antibodies will not exist against the variable structures and first need to develop to mediate full protection.’
Sterilizing immunity
Depending on the overall magnitude and quality of the memory response and the incubation period of the pathogen, it can be eliminated prior to the establishment of the infection. Elimination of the pathogen even before it can infect a host cell and replicate, ideally directly at the site of entry, is referred to as sterilizing immunity (see Box 1 for glossary of terms). Antibodies are the main mediators of sterilizing immunity, but T cells may contribute to the elimination of infected host cells before the pathogen starts to replicate. By definition, sterilizing immunity prevents pathogen transmission. Box 1
Once enough individuals in a population have developed protective immune memory, transmission becomes a rare event because infected individuals are unlikely to meet a non-protected individual while they are contagious. This indirect protection of few non-immune individuals in a population of mostly protected individuals is referred to as herd immunity (Randolph and Barreiro, 2020). Herd immunity also protects those that mount insufficient immune responses or cannot be vaccinated and provides the basis for elimination (regional) and complete eradication (global) of a disease, which has been achieved for smallpox, a once devastating viral disease with high lethality.
The induction of sterilizing immunity is often considered to be the ultimate goal in vaccinology. However, a clear discrimination between sterilizing and protective immunity that prevents symptomatic infection in humans is difficult, if not impossible. Early infections are often asymptomatic, and low levels of pathogen replication in tissues or organs are undetectable. For example, infections with malaria parasites, which first infect and multiply in liver cells, are asymptomatic until thousands of parasites are released into the blood stream and start infecting and multiplying in erythrocytes. The number of infected hepatocytes is low and cannot be measured directly. Therefore, the only indicator for sterilizing immunity is the absence of detectable levels of blood-stage parasites. But even then, it remains unclear whether parasite development was blocked before (antibody-mediated) or after (T cell-mediated) the invasion and multiplication in hepatocytes or by the combined action of B and T cells.
The difficulty in defining sterilizing immunity is not limited to pathogens with complex life cycles. The virus-neutralizing activity of antibodies can be readily defined in robust in vitro assays and in passive transfer experiments. But to what degree vaccination-induced humoral immune responses prevent infection and pathogen replication also in humans, and therefore serve as indicators or even correlates of sterilizing immunity, cannot be easily established. Thus, without measurable parameters that clearly define correlates of sterilizing immunity for every pathogen and disease, the line between protective and sterilizing immunity that truly prevents the infection and pathogen replication is narrow.
Sterilizing immunity and vaccine development
Most vaccines mediate protection through antibodies that directly target structures (antigens) on the pathogen surface (Pollard and Bijker, 2021). By promoting affinity maturation, T cells play a key role in the development of protective antibody responses of high affinity. Vaccines based on replication-deficient (attenuated) pathogens mediate potent immune protection, which is difficult to achieve with protein-based subunit vaccines. Several factors contribute to these differences in efficacy, including the magnitude and breadth of the innate immune response and the amount and stability of the target antigen that determines the length of the vaccine response (Figure 1). Persistent antigen availability or repeated booster immunizations promote the induction of durable immune responses by increasing the quantity and quality of the memory response. Antibody affinity maturation is a key determinant of protective immune memory and mediates changes in the quality of antibody responses with time. The underlying evolutionary process relies on the random mutation-mediated generation of antibody variants and subsequent selection of antibodies with improved antigen-binding properties (Victora and Nussenzweig, 2022). Antigen persistence and booster immunizations that reactivate pre-existing memory cells drive this process and allow the continued maturation of the antibody response over time.
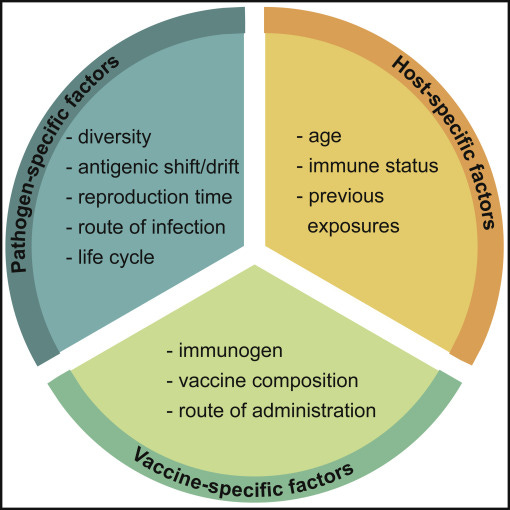
Affinity maturation plays an important role in the development of high-affinity antibodies that interfere directly with the host cell invasion process of intracellular pathogens (neutralizing antibodies) and are therefore the most efficient mediators of sterilizing immunity. Their role is well understood in many viral infections where they block binding of viral surface proteins to host cell receptors, preventing virus entry. Nonetheless, non-neutralizing, high-affinity antibodies contribute to viral clearance and the elimination of extracellular bacterial and parasitic pathogens indirectly via interactions with innate immune molecules and cells.
Pathogen immune evasion
Host-to-host transmission is essential for long-term pathogen survival. To avoid human immune responses that prevent efficient transmission, pathogens have developed various passive and active immune evasion strategies that target innate and adaptive protection mechanisms (Figure 1). These evasion strategies pose great challenges for the development of efficacious vaccines, which protect from disease and prevent transmission to non-immune hosts (Pollard and Bijker, 2021). This is especially true for viral, bacterial, and parasitic pathogens that quickly hide in host cells without replicating and establish chronic latent infections.
Pathogens have developed efficient strategies to escape natural and vaccine-induced immunity. Because adaptive immune responses are characterized by their high specificity, increasing the antigenic diversity is a major immune evasion mechanism. Genetic diversity plays a key role in increasing antigen diversity and can be achieved in different ways. To change their appearance, bacteria and parasites generate antigenic diversity by controlled changes in their gene expression profiles, whereas viruses mutate (antigenic drift) or shuffle (antigenic shift) their genome (Yewdell, 2021). While antigenic drift is achieved by an error-prone replication of the viral genome, antigenic shift, as seen for influenza virus, is mediated by the exchange of genome fragments during co-infection of the same host cell with two distinct viruses. Large viral reservoirs and transmission events from animals increase the chance of antigenic shift events.
However, pathogen evolution is also influenced by the host immune response. Antibodies strongly drive the selection of variants that are able to escape the immune pressure exerted by the host immune system. In infections with human immunodeficiency virus (HIV), a virus that is characterized by its high mutation rate and diverse immune evasion strategies, this co-evolution process can be followed longitudinally in a single infected individual (Bonsignori et al., 2017). Humoral immune responses usually fail to control HIV infection, as the high mutation rate of HIV results in the fast emergence of viral variants escaping antibody recognition. However, antibodies targeting conserved epitopes of functional relevance for the virus can develop over time and show activity against newly emerging variants. Similar to the virus that they target, these broadly neutralizing antibodies show strong signs of evolution through mutations and concomitant selection as part of the natural affinity maturation process that underlies the development of high-affinity memory antibodies (Victora and Nussenzweig, 2022). The development of broadly neutralizing antibodies is not limited to HIV, demonstrating the potential of the humoral immune system to overcome antigenic diversity (Corti and Lanzavecchia, 2013). Nevertheless, the development of vaccines that induce broadly neutralizing antibody responses remains a challenge (Pollard and Bijker, 2021).
The SARS-CoV-2 pandemic
The ongoing SARS-CoV-2 pandemic illustrates the host-pathogen co-evolution at population level and is a textbook example of the basic principles that underlie immune protection and neutralizing antibody responses. The initial lack of pre-existing immunity at the beginning of the pandemic allowed the virus to quickly spread globally, causing millions of deaths. Fortunately, numerous highly efficacious vaccines against the original virus were developed at unprecedented speed, including potent mRNA-based subunit vaccines that mediated about 95% protection from COVID-19 after only two immunizations. Protection was associated with the induction of strong neutralizing serum antibody responses against the receptor binding domain (RBD) of the spike (S) protein on the virus surface that mediates infection of the host cell. Efficacy relied on high serum antibody levels, likely because the vaccine-induced systemic immune response produces immunoglobulin G (IgG) antibodies that are not efficiently transported across the epithelial barrier in the upper respiratory tract to mediate neutralization at the site of virus entry.
Although the SARS-CoV-2 mutation rate is low, so many people were infected that the random development of mutants with survival advantages in any of the millions of infected individuals worldwide was just a matter of time. Large sequencing efforts allowed live monitoring of the viral genome evolution throughout the course of the pandemic. Due to their improved transmission properties, some of the newly emerging variants (α, β, γ, δ) spread quickly, wiped out the original virus, and dominated the global infection process in waves (Callaway, 2021). The increasing immune pressure induced by natural and vaccine-induced immunity promoted the selection of the now-dominant omicron variant with numerous mutations in the spike RBD that mediate immune escape from humoral immunity. The evolutionary selection process continues, as evidenced by the continued global spreading of new omicron subvariants. Despite the clear lack of sterilizing immunity against omicron, memory induced by infection with the wild-type virus or vaccination mediates strong protection from severe disease compared with non-vaccinated individuals without prior SARS-CoV-2 memory (Figure 2).
Cross-protection is likely mediated by rare broadly neutralizing antibodies against areas of the RBD with limited antigenic diversity (Wang et al., 2021), although CD8+ T cells specific for conserved regions within any of the viral proteins likely contribute to immunity against the variants. By reducing the viral burden, cross-protective immunity helps limit transmission and the emergence of novel viral escape variants.
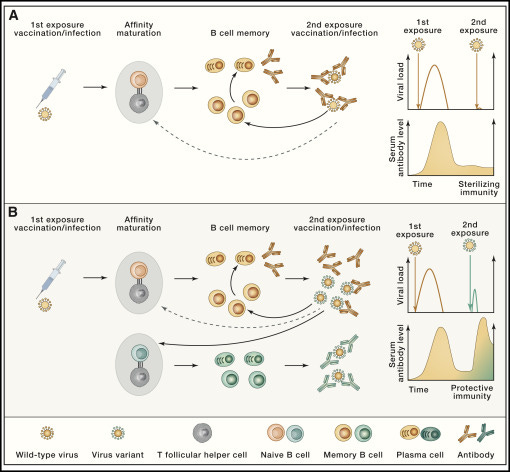
Parallel to the activation of pre-existing memory cells against conserved epitopes that will initially dominate the anti-variant response, specific B cell responses against non-conserved epitopes will be induced. Because these cells need to undergo affinity maturation and expansion to form memory, they will be less frequent and require repeated exposures to build up memory against variant-specific epitopes. Nevertheless, they will broaden the response and thereby contribute to protection.
Challenges for the induction of sterilizing immunity against SARS-CoV-2
Although highly efficacious vaccines against COVID-19 were developed within less than a year, they were not distributed equally across the world. Low vaccination rates facilitate the global spreading of newly emerging SARS-CoV-2 variants, causing severe disease among non-vaccinated individuals. Because the virus relies on interactions with host receptors for cell invasion, the mutation space may be limited by the virus’ need to balance transmissibility with escape from humoral immunity. Importantly, the new mRNA-based vaccine platforms allow for the rapid development of adapted vaccines against selected variants such as omicron. Although new viral variants will likely continue to emerge, pre-existing antibodies and memory B and T cells induced by vaccination against the original virus or prior infections that target conserved epitopes will contribute to cross-protection from severe disease and death (Wang et al., 2021).
Nevertheless, sterilizing immunity will be difficult to achieve unless viral evolution slows down or vaccination and infection-mediated immunity induce highly efficacious broadly neutralizing antibody responses (Reynolds et al., 2021). Strong humoral responses in the upper respiratory tract might be necessary for protection from the infection at the site of virus entry, but potent mucosal immunity is not easy to achieve by vaccination. Nonetheless, even in the absence of sterilizing immunity, immune memory will not only protect from disease, but also help reduce transmission and the size of the circulating virus pool.
Recap
Antibody-mediated sterilizing immunity is the most effective way of the adaptive immune system to defeat invading pathogens by preventing the establishment of the infection. Repeated vaccinations or pathogen exposures can induce sterilizing immune memory, but through antigenic diversity, pathogens escape sterilizing immunity. The ongoing SARS-CoV-2 pandemic exemplifies this pathogen-host co-evolution process on a global scale. The fast transmission of SARS-CoV-2 facilitated the development of a large virus reservoir and the concomitant generation of random variants that continue to be selected for higher transmissibility and immune escape properties.
The spreading of the omicron variant with numerous mutations that mediate immune escape from neutralizing antibodies reflects this process. Although all available vaccines were developed against a virus that was quickly replaced, they show sufficient breadth to protect efficiently from severe COVID-19 caused by any of the SARS-CoV-2 variants, including omicron. Thus, despite the absence of sterilizing immunity, COVID-19 vaccination saved millions of lives, likely mediated by cross-protective antibodies and T cells against conserved epitopes. To end the pandemic, the goal is to reduce the size of the viral reservoir. Natural and vaccine-induced immunity will help to reduce transmission, thereby lowering the likelihood for the development and spread of novel escape variants.